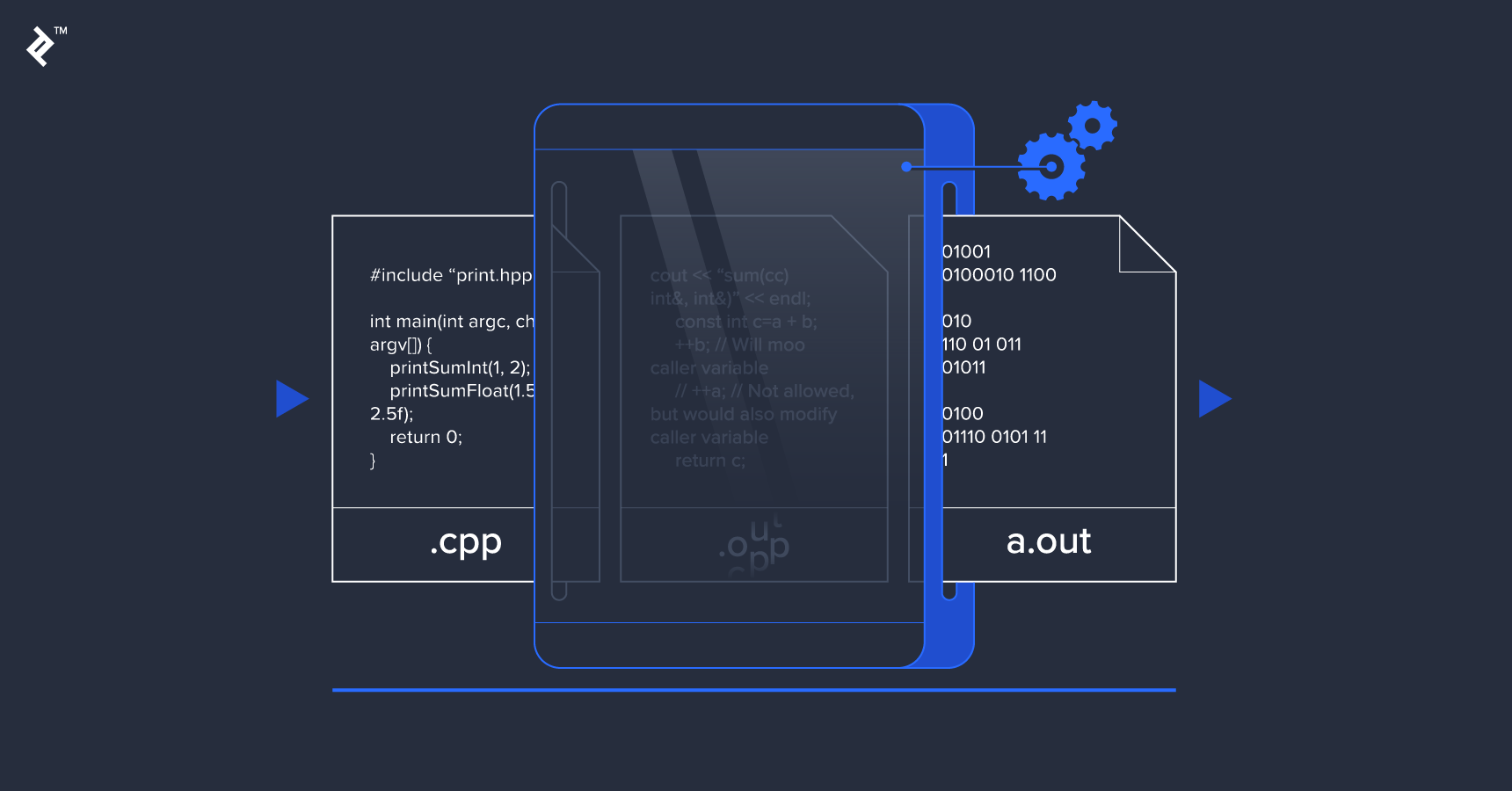
How C++ Works: Understanding Compilation | Toptal
How C++ Works: Understanding Compilation
Daniel has created high-performance applications in C++ for large companies such as Dreamworks. He also excels with C and ASM (x86).
57SHARES
Bjarne Stroustrup’s The C++ Programming Language has a chapter titled “A Tour of C++: The Basics”—Standard C++. That chapter, in 2.2, mentions in half a page the compilation and linking process in C++. Compilation and linking are two very basic processes that happen all the time during C++ software development, but oddly enough, they aren’t well understood by many C++ developers.
Why is C++ source code split into header and source files? How is each part seen by the compiler? How does that affect compilation and linking? There are many more questions like these that you may have thought about but have come to accept as convention.
Whether you are designing a C++ application, implementing new features for it, trying to address bugs (especially certain strange bugs), or trying to make C and C++ code work together, knowing how compilation and linking works will save you a lot of time and make those tasks much more pleasant. In this article, you will learn exactly that.
The article will explain how a C++ compiler works with some of the basic language constructs, answer some common questions that are related to their processes, and help you work around some related mistakes that developers often make in C++ development.
Note: This article has some example source code that can be downloaded from https://bitbucket.org/danielmunoz/cpp-article
The examples were compiled in a CentOS Linux machine:
$ uname -srLinux 3.10.0-327.36.3.el7.x86_64
Using g++ version:
$ g++ --versiong++ (GCC) 4.8.5 20150623 (Red Hat 4.8.5-11)
The source files provided should be portable to other operating systems, although the Makefiles accompanying them for the automated build process should be portable only to Unix-like systems.
The Build Pipeline: Preprocess, Compile, and Link
Each C++ source file needs to be compiled into an object file. The object files resulting from the compilation of multiple source files are then linked into an executable, a shared library, or a static library (the last of these being just an archive of object files). C++ source files generally have the .cpp, .cxx or .cc extension suffixes.
A C++ source file can include other files, known as header files, with the #include directive. Header files have extensions like .h, .hpp, or .hxx, or have no extension at all like in the C++ standard library and other libraries’ header files (like Qt). The extension doesn’t matter for the C++ preprocessor, which will literally replace the line containing the #include directive with the entire content of the included file.
The first step that the compiler will do on a source file is run the preprocessor on it. Only source files are passed to the compiler (to preprocess and compile it). Header files aren’t passed to the compiler. Instead, they are included from source files.
Each header file can be opened multiple times during the preprocessing phase of all source files, depending on how many source files include them, or how many other header files that are included from source files also include them (there can be many levels of indirection). Source files, on the other hand, are opened only once by the compiler (and preprocessor), when they are passed to it.
For each C++ source file, the preprocessor will build a translation unit by inserting content in it when it finds an #include directive at the same time that it’ll be stripping code out of the source file and of the headers when it finds conditional compilation blocks whose directive evaluates to false. It’ll also do some other tasks like macro replacements.
Once the preprocessor finishes creating that (sometimes huge) translation unit, the compiler starts the compilation phase and produces the object file.
To obtain that translation unit (the preprocessed source code), the -E option can be passed to the g++ compiler, along with the -o option to specify the desired name of the preprocessed source file.
In the cpp-article/hello-world directory, there is a “hello-world.cpp” example file:
#include <iostream>int main(int argc, char* argv[]) {std::cout << "Hello world" << std::endl;return 0;}
Create the preprocessed file by:
$ g++ -E hello-world.cpp -o hello-world.ii
And see the number of lines:
$ wc -l hello-world.ii17558 hello-world.ii
It has 17,588 lines in my machine. You can also just run make on that directory and it’ll do those steps for you.
We can see that the compiler must compile a much larger file than the simple source file that we see. This is because of the included headers. And in our example, we have included just one header. The translation unit becomes bigger and bigger as we keep including headers.
This preprocess and compile process is similar for C language. It follows the C rules for compiling, and the way it includes header files and produces object code is nearly the same.
How Source Files Import and Export Symbols
Let’s see now the files in cpp-article/symbols/c-vs-cpp-names directory.
There is a simple C (not C++) source file named sum.c that exports two functions, one for adding two integers and one for adding two floats:
int sumI(int a, int b) {return a + b;}float sumF(float a, float b) {return a + b;}
Compile it (or run make and all the steps to create the two example apps to be executed) to create the sum.o object file:
$ gcc -c sum.c
Now look at the symbols exported and imported by this object file:
$ nm sum.o0000000000000014 T sumF0000000000000000 T sumI
No symbols are imported and two symbols are exported: sumF and sumI. Those symbols are exported as part of the .text segment (T), so they are function names, executable code.
If other (both C or C++) source files want to call those functions, they need to declare them before calling.
The standard way to do it is to create a header file that declares them and includes them in whatever source file we want to call them. The header can have any name and extension. I chose sum.h:
#ifdef __cplusplusextern "C" {#endifint sumI(int a, int b);float sumF(float a, float b);#ifdef __cplusplus} // end extern "C"#endif
What are those ifdef/endif conditional compilation blocks? If I include this header from a C source file, I want it to become:
int sumI(int a, int b);float sumF(float a, float b);
But if I include them from a C++ source file, I want it to become:
extern "C" {int sumI(int a, int b);float sumF(float a, float b);} // end extern "C"
C language doesn’t know anything about the extern "C" directive, but C++ does, and it needs this directive applied to C function declarations. This is because C++ mangles function (and method) names because it supports function/method overloading, while C doesn’t.
This can be seen in the C++ source file named print.cpp:
#include <iostream> // std::cout, std::endl#include "sum.h" // sumI, sumFvoid printSum(int a, int b) {std::cout << a << " + " << b << " = " << sumI(a, b) << std::endl;}void printSum(float a, float b) {std::cout << a << " + " << b << " = " << sumF(a, b) << std::endl;}extern "C" void printSumInt(int a, int b) {printSum(a, b);}extern "C" void printSumFloat(float a, float b) {printSum(a, b);}
There are two functions with the same name (printSum) that only differ in their parameters’ type: int or float. Function overloading is a C++ feature which isn’t present in C. To implement this feature and differentiate those functions, C++ mangles the function name, as we can see in their exported symbol name (I’ll only pick what’s relevant from nm’s output):
$ g++ -c print.cpp$ nm print.o0000000000000132 T printSumFloat0000000000000113 T printSumIntU sumFU sumI0000000000000074 T _Z8printSumff0000000000000000 T _Z8printSumiiU _ZSt4cout
Those functions are exported (in my system) as _Z8printSumff for the float version and _Z8printSumii for the int version. Every function name in C++ is mangled unless declared as extern "C". There are two functions that were declared with C linkage in print.cpp: printSumInt and printSumFloat.
Therefore, they cannot be overloaded, or their exported names would be the same since they aren’t mangled. I had to differentiate them from each other by postfixing an Int or a Float to the end of their names.
Since they are not mangled they can be called from C code, as we’ll soon see.
To see the mangled names like we would see them in C++ source code, we can use the -C (demangle) option in the nm command. Again, I’ll only copy the same relevant part of the output:
$ nm -C print.o0000000000000132 T printSumFloat0000000000000113 T printSumIntU sumFU sumI0000000000000074 T printSum(float, float)0000000000000000 T printSum(int, int)U std::cout
With this option, instead of _Z8printSumff we see printSum(float, float), and instead of _ZSt4cout we see std::cout, which are more human-friendly names.
We also see that our C++ code is calling C code: print.cpp is calling sumI and sumF, which are C functions declared as having C linkage in sum.h. This can be seen in the nm output of print.o above, that informs of some undefined (U) symbols: sumF, sumI and std::cout. Those undefined symbols are supposed to be provided in one of the object files (or libraries) that will be linked together with this object file output in the link phase.
So far we have just compiled source code into object code, we haven’t yet linked. If we don’t link the object file that contains the definitions for those imported symbols together with this object file, the linker will stop with a “missing symbol” error.
Note also that since print.cpp is a C++ source file, compiled with a C++ compiler (g++), all the code in it is compiled as C++ code. Functions with C linkage like printSumInt and printSumFloat are also C++ functions that can use C++ features. Only the names of the symbols are compatible with C, but the code is C++, which can be seen by the fact that both functions are calling an overloaded function (printSum), which couldn’t happen if printSumInt or printSumFloat were compiled in C.
Let’s see now print.hpp, a header file that can be included both from C or C++ source files, which will allow printSumInt and printSumFloat to be called both from C and from C++, and printSum to be called from C++:
#ifdef __cplusplusvoid printSum(int a, int b);void printSum(float a, float b);extern "C" {#endifvoid printSumInt(int a, int b);void printSumFloat(float a, float b);#ifdef __cplusplus} // end extern "C"#endif
If we are including it from a C source file, we just want to see:
void printSumInt(int a, int b);void printSumFloat(float a, float b);
printSum can’t be seen from C code since its name is mangled, so we don’t have a (standard and portable) way to declare it for C code. Yes, I can declare them as:
void _Z8printSumii(int a, int b);void _Z8printSumff(float a, float b);
And the linker won’t complain since that’s the exact name that my currently installed compiler invented for it, but I don’t know if it’ll work for your linker (if your compiler generates a different mangled name), or even for the next version of my linker. I don’t even know if the call will work as expected because of the existence of different calling conventions (how parameters are passed and return values are returned) that are compiler specific and may be different for C and C++ calls (especially for C++ functions that are member functions and receive the this pointer as a parameter).
Your compiler can potentially use one calling convention for regular C++ functions and a different one if they are declared as having extern “C” linkage. So, cheating the compiler by saying that one function uses C calling convention while it actually uses C++ for it can deliver unexpected results if the conventions used for each happen to be different in your compiling toolchain.
There are standard ways to mix C and C++ code and a standard way to call C++ overloaded functions from C is to wrap them in functions with C linkage as we did by wrapping printSum with printSumInt and printSumFloat.
If we include print.hpp from a C++ source file, the __cplusplus preprocessor macro will be defined and the file will be seen as:
void printSum(int a, int b);void printSum(float a, float b);extern "C" {void printSumInt(int a, int b);void printSumFloat(float a, float b);} // end extern "C"
This will allow C++ code to call the overloaded function printSum or its wrappers printSumInt and printSumFloat.
Now let’s create a C source file containing the main function, which is the entry point for a program. This C main function will call printSumInt and printSumFloat, that is, will call both C++ functions with C linkage. Remember, those are C++ functions (their function bodies execute C++ code) that only don’t have C++ mangled names. The file is named c-main.c:
#include "print.hpp"int main(int argc, char* argv[]) {printSumInt(1, 2);printSumFloat(1.5f, 2.5f);return 0;}
Compile it to generate the object file:
$ gcc -c c-main.c
And see the imported/exported symbols:
$ nm c-main.o0000000000000000 T mainU printSumFloatU printSumInt
It exports main and imports printSumFloat and printSumInt, as expected.
To link it all together into an executable file, we need to use the C++ linker (g++), since at least one file that we’ll link, print.o, was compiled in C++:
$ g++ -o c-app sum.o print.o c-main.o
The execution produces the expected result:
$ ./c-app1 + 2 = 31.5 + 2.5 = 4
Now let’s try with a C++ main file, named cpp-main.cpp:
#include "print.hpp"int main(int argc, char* argv[]) {printSum(1, 2);printSum(1.5f, 2.5f);printSumInt(3, 4);printSumFloat(3.5f, 4.5f);return 0;}
Compile and see the imported/exported symbols of the cpp-main.o object file:
$ g++ -c cpp-main.cpp$ nm -C cpp-main.o0000000000000000 T mainU printSumFloatU printSumIntU printSum(float, float)U printSum(int, int)
It exports main and imports C linkage printSumFloat and printSumInt, and both mangled versions of printSum.
You may be wondering why the main symbol isn’t exported as a mangled symbol like main(int, char**) from this C++ source since it’s a C++ source file and it isn’t defined as extern "C". Well, main is a special implementation defined function and my implementation seems to have chosen to use C linkage for it no matter whether it’s defined in a C or C++ source file.
Linking and running the program gives the expected result:
$ g++ -o cpp-app sum.o print.o cpp-main.o$ ./cpp-app1 + 2 = 31.5 + 2.5 = 43 + 4 = 73.5 + 4.5 = 8
How Header Guards Work
So far, I’ve been careful not to include my headers twice, directly or indirectly, from the same source file. But since one header can include other headers, the same header can indirectly be included multiple times. And since header content is just inserted in the place from where it was included, it’s easy to end with duplicated declarations.
See the example files in cpp-article/header-guards.
// unguarded.hppclass A {public:A(int a) : m_a(a) {}void setA(int a) { m_a = a; }int getA() const { return m_a; }private:int m_a;};// guarded.hpp:#ifndef __GUARDED_HPP#define __GUARDED_HPPclass A {public:A(int a) : m_a(a) {}void setA(int a) { m_a = a; }int getA() const { return m_a; }private:int m_a;};#endif // __GUARDED_HPP
The difference is that, in guarded.hpp, we surround the entire header in a conditional that will only be included if __GUARDED_HPP preprocessor macro isn’t defined. The first time that the preprocessor includes this file, it won’t be defined. But, since the macro is defined inside that guarded code, the next time it’s included (from the same source file, directly or indirectly), the preprocessor will see the lines between the #ifndef and the #endif and will discard all the code between them.
Note that this process happens for every source file that we compile. It means that this header file can be included once and only once for each source file. The fact that it was included from one source file won’t prevent it to be included from a different source file when that source file is compiled. It’ll just prevent it to be included more than once from the same source file.
The example file main-guarded.cpp includes guarded.hpp twice:
#include "guarded.hpp"#include "guarded.hpp"int main(int argc, char* argv[]) {A a(5);a.setA(0);return a.getA();}
But the preprocessed output only shows one definition of class A:
$ g++ -E main-guarded.cpp# 1 "main-guarded.cpp"# 1 "<built-in>"# 1 "<command-line>"# 1 "/usr/include/stdc-predef.h" 1 3 4# 1 "<command-line>" 2# 1 "main-guarded.cpp"# 1 "guarded.hpp" 1class A {public:A(int a) : m_a(a) {}void setA(int a) { m_a = a; }int getA() const { return m_a; }private:int m_a;};# 2 "main-guarded.cpp" 2int main(int argc, char* argv[]) {A a(5);a.setA(0);return a.getA();}
Therefore, it can be compiled without problems:
$ g++ -o guarded main-guarded.cpp
But the main-unguarded.cpp file includes unguarded.hpp twice:
#include "unguarded.hpp"#include "unguarded.hpp"int main(int argc, char* argv[]) {A a(5);a.setA(0);return a.getA();}
And the preprocessed output shows two definitions of class A:
$ g++ -E main-unguarded.cpp# 1 "main-unguarded.cpp"# 1 "<built-in>"# 1 "<command-line>"# 1 "/usr/include/stdc-predef.h" 1 3 4# 1 "<command-line>" 2# 1 "main-unguarded.cpp"# 1 "unguarded.hpp" 1class A {public:A(int a) : m_a(a) {}void setA(int a) { m_a = a; }int getA() const { return m_a; }private:int m_a;};# 2 "main-unguarded.cpp" 2# 1 "unguarded.hpp" 1class A {public:A(int a) : m_a(a) {}void setA(int a) { m_a = a; }int getA() const { return m_a; }private:int m_a;};# 3 "main-unguarded.cpp" 2int main(int argc, char* argv[]) {A a(5);a.setA(0);return a.getA();}
This will cause problems when compiling:
$ g++ -o unguarded main-unguarded.cpp
In file included from main-unguarded.cpp:2:0:
unguarded.hpp:1:7: error: redefinition of 'class A'class A {^In file included from main-unguarded.cpp:1:0:unguarded.hpp:1:7: error: previous definition of 'class A'class A {^
For the sake of brevity, I won’t use guarded headers in this article if it isn’t necessary since most are short examples. But always guard your header files. Not your source files, which won’t be included from anywhere. Just header files.
Pass by Value and Constness of Parameters
Look at by-value.cpp file in cpp-article/symbols/pass-by:
#include <vector>#include <numeric>#include <iostream>// std::vector, std::accumulate, std::cout, std::endlusing namespace std;int sum(int a, const int b) {cout << "sum(int, const int)" << endl;const int c = a + b;++a; // Possible, not const// ++b; // Not possible, this would result in a compilation errorreturn c;}float sum(const float a, float b) {cout << "sum(const float, float)" << endl;return a + b;}int sum(vector<int> v) {cout << "sum(vector<int>)" << endl;return accumulate(v.begin(), v.end(), 0);}float sum(const vector<float> v) {cout << "sum(const vector<float>)" << endl;return accumulate(v.begin(), v.end(), 0.0f);}
Since I use the using namespace std directive, I don’t have to qualify the names of symbols (functions or classes) inside the std namespace in the rest of the translation unit, which in my case is the rest of the source file. If this were a header file, I shouldn’t have inserted this directive because a header file is supposed to be included from multiple source files; this directive would bring to the global scope of each source file the entire std namespace from the point they include my header.
Even headers included after mine in those files will have those symbols in scope. This can produce name clashes since they were not expecting this to happen. Therefore, don’t use this directive in headers. Only use it in source files if you want, and only after you included all headers.
Note how some parameters are const. This means that they can’t be changed in the body of the function if we try to. It’d give a compilation error. Also, note that all the parameters in this source file are passed by value, not by reference (&) or by pointer (*). This means that the caller will make a copy of them and pass to the function. So, it doesn’t matter for the caller whether they are const or not, because if we modify them in the function body we’ll only be modifying the copy, not the original value the caller passed to the function.
Since the constness of a parameter that is passed by value (copy) doesn’t matter for the caller, it is not mangled in the function signature, as it can be seen after compiling and inspecting the object code (only the relevant output):
$ g++ -c by-value.cpp$ nm -C by-value.o000000000000001e T sum(float, float)0000000000000000 T sum(int, int)0000000000000087 T sum(std::vector<float, std::allocator<float> >)0000000000000048 T sum(std::vector<int, std::allocator<int> >)
The signatures don’t express whether the copied parameters are const or not in the bodies of the function. It doesn’t matter. It mattered for the function definition only, to show at a glance to the reader of the function body whether those values will ever change. In the example, only half of the parameters are declared as const, so we can see the contrast, but if we want to be const-correct they should all have been declared so since none of them are modified in the function body (and they shouldn’t).
Since it doesn’t matter for the function declaration which is what the caller sees, we can create the by-value.hpp header like this:
#include <vector>int sum(int a, int b);float sum(float a, float b);int sum(std::vector<int> v);int sum(std::vector<float> v);
Adding the const qualifiers here is allowed (you can even qualify as const variables that aren’t const in the definition and it’ll work), but this is not necessary and it’ll only make the declarations unnecessarily verbose.
Pass by Reference
Let’s see by-reference.cpp:
#include <vector>#include <iostream>#include <numeric>using namespace std;int sum(const int& a, int& b) {cout << "sum(const int&, int&)" << endl;const int c = a + b;++b; // Will modify caller variable// ++a; // Not allowed, but would also modify caller variablereturn c;}float sum(float& a, const float& b) {cout << "sum(float&, const float&)" << endl;return a + b;}int sum(const std::vector<int>& v) {cout << "sum(const std::vector<int>&)" << endl;return accumulate(v.begin(), v.end(), 0);}float sum(const std::vector<float>& v) {cout << "sum(const std::vector<float>&)" << endl;return accumulate(v.begin(), v.end(), 0.0f);}
Constness when passing by reference matters for the caller, because it’ll tell the caller whether its argument will be modified or not by the callee. Therefore, the symbols are exported with their constness:
$ g++ -c by-reference.cpp$ nm -C by-reference.o0000000000000051 T sum(float&, float const&)0000000000000000 T sum(int const&, int&)00000000000000fe T sum(std::vector<float, std::allocator<float> > const&)00000000000000a3 T sum(std::vector<int, std::allocator<int> > const&)
That should also be reflected in the header that callers will use:
#include <vector>int sum(const int&, int&);float sum(float&, const float&);int sum(const std::vector<int>&);float sum(const std::vector<float>&);
Note that I didn’t write the name of the variables in the declarations (in the header) as I’d been doing so far. This is also legal, for this example and for the previous ones. Variable names aren’t required in the declaration, since the caller doesn’t need to know how do you want to name your variable. But parameter names are generally desirable in declarations so the user can know at a glance what each parameter mean and therefore what to send in the call.
Surprisingly, variable names aren’t either needed in the definition of a function. They are only needed if you actually use the parameter in the function. But if you never use it you can leave the parameter with the type but without the name. Why would a function declare a parameter that it’d never use? Sometimes functions (or methods) are just part of an interface, like a callback interface, which defines certain parameters that are passed to the observer. The observer must create a callback with all the parameters that the interface specifies since they’ll be all sent by the caller. But the observer may not be interested in all of them, so instead of receiving a compiler warning about an “unused parameter,” the function definition can just leave it without a name.